Storing Emissions Underground (Part 1)
We now have the geologic storage options we need to scale up carbon capture
You’re reading Terraform Now, my newsletter on carbon capture and other planet-altering technologies. To support my work, you can subscribe here:
TLDR:
Storing CO2 underground is a better long-term option than using CO2 for fuels, fertilizer, etc. These ‘utilization’ markets are too small
The best storage caverns are large, >800m underground, and have a caprock that seals in CO2
Three rock formations are proven to work for CO2: empty oil wells, salty underground lakes called aquifers, and basalt formations created by cooling lava
Many observers of carbon capture say the ‘exciting’ part of the process is the technology that actually captures CO2 out of the sky, while storage is considered less interesting. As Matt Yglesias has written:
The “hole in the ground” part of carbon capture technology is clearly not the interesting part. What’s interesting is the efforts to capture carbon dioxide in the first place.
I couldn’t disagree more with this — I think geologic storage is mind-boggling and mysterious. Imagining how we compress a few million tons of gas into a liquid, and pump it into a giant, dark cavern half a mile underground is a bit like wrapping my head around the distance between the Earth and the Moon. There are numbers, there are words to describe it, but our minds balk at the depth, darkness, and scale of it all.
You can have different reactions to the mystery of CO2 storage. You can get bored and move on. You can be curious and want to learn more (keep reading if you’re in this group). Or, you can be intimidated by the awe-inspiring engineering and conclude that storage must incredibly dangerous, then use incendiary political tactics to hold up storage projects.
Folks in that last camp are often well-meaning environmentalists, but they misunderstand the science and end up holding back progress on the climate transition. Storage is important, safe, and we need to do it.
WHY STORAGE MATTERS
We need both Direct Air Capture (DAC) and Emissions’ Source Carbon Capture to manage the climate transition. If you think that moving to renewables energy alone will keep global temperatures low, I’d suggest you check out Terraform Now’s The Case for Carbon Capture.
TLDR: carbon capture is the best technology we have to get rid of historic greenhouse gas emissions, and is a good insurance policy against higher-than-expected future emissions, which are very likely.
More than 99% of the CO2 we capture via DAC or Emissions’ source tech will need to be locked away permanently, and right now storing CO2 underground is the most mature technology for permanent storage.
One thing that makes geologic storage so attractive is how much of it there is. There is plenty of space underground to store all of our historic and future emissions underground:
As I wrote in Terraform Now’s Carbon Capture Primer:
The high-end estimate of geologic storage that can safely store CO2 is ~16 trillion tons. The amount of CO2 humans emitted since 1850, plus the amount we will emit from 2023 until 2050 is ~2.1 trillion tons — so storage capacity could outpace the CO2 we need to capture by ~8x.
If you want to be pessimistic and believe the low-end storage estimates, it will be a very long time before we need to worry about running out of storage. Today we are only capturing 45 million tons of CO2 per year, less than 0.001% of the ~1.9 trillion ton low-end estimate.
So there’s a lot of space to put CO2 underground — that’s great! But burying it underground seems like a crude solution, and many people are figuring out ways to use CO2 for something productive — fertilizing crops, carbonating soft drinks, etc. Unfortunately, none of these so-called ‘utilization’ markets is big enough to scale with carbon capture. In total about ~300 million tons of CO2 are used per year, and even though that sounds like a lot, it’s <1% of global CO2 emissions.
None of these use cases are growing quickly. As you can imagine, things like fertilizer are mature markets growing at no more than 3% per year. To believe in utilization, you have to believe that new ways to use are going to grow very fast in the coming years decades.
I’m bullish on one new use case for CO2, recycled fuels, also called drop-in fuels. Companies like Porsche are combining CO2 and industrial by-products to create fuels that can be used in existing cars and trucks. These fuels are ‘drop-in’ because we don’t have to change anything about the cars and trucks, we can just replace the fuel.
Recycled fuels are gaining some momentum, but even if they are widely adopted, recycled fuels are unlikely to ever consume more than 5% or 10% of the CO2 that we capture1. You can make same arguments for other new use cases — from carbon-infused cement to mass-produced graphene.
So, storage is the best option because, barring miraculous new use cases for CO2, it is the only option that can scale with the amount of CO2 we’re going to want to capture. So how are we actually going to store it all?
STORAGE OPTIONS
The geography of storage sites will decide the location of large DAC facilities. These can be built anywhere, since they are sucking CO2 from the atmosphere, but building them on top of good geologic storage sites cuts out transportation cost and a multi-year permitting process for pipelines.
Geologists look for a few things in a storage site:
Empty space: caverns, arteries, and pockets where CO2 can settle. One big cavern is better than many small ones, because there large fixed costs of developing a storage site
Sealing rock: solid layers of rock between the cavern and the surface keeps CO2 from leaking out. Often called a caprock when drilling oil, for CO2 storage many different formations will work
Deep underground: caverns should be at least 800 meters deep. This creates enough pressure to keep CO2 in liquid form, keeps CO2 away from drinking water, and reduces leakage to the surface
Generally speaking, there are three types of rock that have all of these things: old oil and gas wells, salty underground lakes called aquifers, and basalt formations created by cooling lava. There are a bunch of other formations that scientists are trying, but these three are proven options that can meet the needs of a scaled up carbon capture industry all on their own.
FIRST WE’LL FILL UP THE OIL & GAS WELLS
Believe it or not, some oil companies already have experience pumping CO2 down wells. In 2022 they pumped ~80 million tons of CO2 into oil wells that are almost dry. The pressure from incoming CO2 pushes the last bits of oil up and out of the well, and during this pumping process, the CO2 stays trapped underground2.
More broadly, storing CO2 is the ‘mirror image’ of extracting oil from the ground, according to carbon capture expert Howard Herzog. Liquid CO2 behaves a lot like the hydrocarbons that are the basis for crude oil, which means that many of same skills involved in extracting oil — geology, fluid dynamics, chemical engineering — will propel CO2 storage too.
And it’s good that these skills exist, because making old oil & gas wells into usable storage caverns is not easy. For example, old oil projects have not one, but many wells leading to a single cavern. Every single well, big or small, has to be stopped up to prevent seapage to the surface.
There are over one million open wells in Texas alone, so this is no small task. Not only do all these wells have to be plugged, but regulating bodies like the EPA have to be 100% confident that they are plugged. Convincing regulators might be a bigger challenge than the engineering challenge of filling in the wells.
Another way in which storage is a mirror image of oil drilling has to do with pressure. When we take oil out of the ground, we take advantage of a difference in pressure — the pressure in the oil-filled cavern is higher than the pressure on the surface, and so the oil wants to escape up into the pipe and to the surface.
When engineers want to store CO2 underground, they reverse this pressure difference. There must be more pressure in the pipe than in the cavern, so that the CO2 comes out of the pipe quickly to spread throughout the cavern. The caverns are very far underground, which means a lot of rock putting pressure on the cavern, so the pipeline pressure has to be quite high — between 100-150 bar, or 100-150x the pressure of our atmosphere.
But wait, you might ask — I thought that these were empty caverns, so intuitively we wouldn’t need much pressure to ensure that the CO2 is spreading out. Well, these caverns are empty of oil, but they aren’t empty altogether. There’s leftover salty, briny water that forms large underground lakes that we call aquifers.
DEALING WITH AQUIFERS
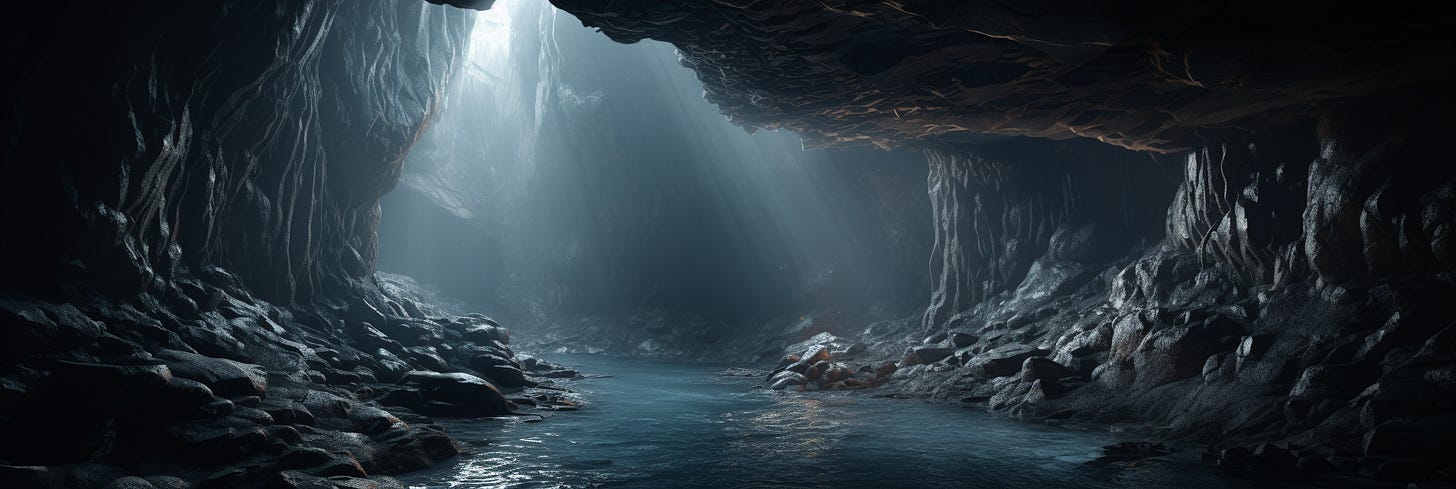
This leftover, briny water is very hard to deal with. It’s salty to the point of being poisonous, and when it seeps up to the surface or into our drinking water it’s bad news for crops and people. After taking out the oil, the reduced pressure in some of these caverns make them a bit unstable, prone to leakage or even collapse, which could cause an earthquake.
For the purposes of storing CO2, though, this instability might be a good thing, since pumping CO2 back down the wells re-pressurizes them to where they were before we removed the oil. Since the oil and water sat in those caverns at that higher pressure for millions of years, we can be fairly confident that CO2 and water at similar pressures won’t escape.
However, the number of old oil wells we can fill up is limited. Ultimately, we’re going to want to find other briny aquifers that never had oil in them. Geologic surveys tell us that there are a lot of briny aquifers without oil. These have much, much more capacity than empty oil wells.
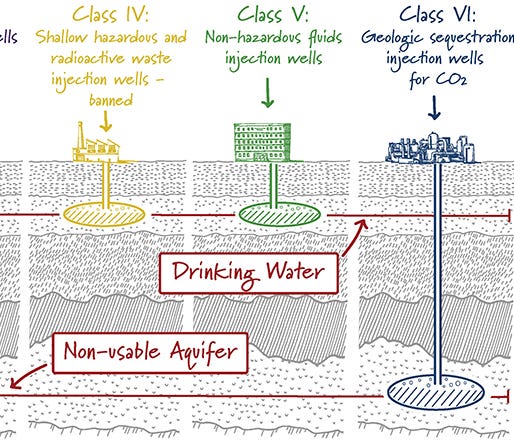
Beginning sometime in the 2030s, as carbon capture scales up, briny aquifers will be an excellent place for us to put CO2. By the time we have moved from old wells to untouched aquifers, we’ll have an solid grasp on the behavior of CO2 in the briny deep because we’ll have dealt with similar situations in the old wells. By then there will probably be a whole sub-profession within geology that deals with the interaction between different types of briny water, rocks, and and CO2.
We might call these future storage professionals Plume Engineers, because tracking the paths the CO2 takes through the cavern, known as plumes, will be the trickiest part of storing CO2 in aquifers. Some plumes will be simple. For example, in some aquifers the CO2 will dissolve into the briny water, making the heavier CO2 infused water drift to the bottom of the aquifer. This circulates more water to the top of the aquifer to take on more CO2.
Other plumes will behave in more complex ways, depending on their exact chemistry, geology, pressure differentials, and shape of the cavern. A plume may go sideways into capillaries that are parallel to the pipe, or up into pockets above the cavern called catchment areas. Whatever paths the CO2 takes, it should be continually monitored and storage plans may change based on where the primary plumes are in the cavern.
BASALT FORMATIONS
After lava comes flowing out of volcanos, it interacts with the atmosphere and begins to cool off. With Earth’s atmosphere (and Earth’s magma), this interaction creates a rock known as basalt, which is full of many little air pockets:
Basalt storage involves filling those air pockets with liquid carbon dioxide. What makes this approach so exciting is that the CO2 will solidify and become part of the rock itself, a process known as mineralization. Carbfix, a company that has pumped almost 100,000 tons of CO2 into basalt formations, has a great primer on this process:
Carbonated water is acidic. The more carbon you can pack into water, the more acidic the fluid will become. Carbfix's carbonated water reacts with rocks underground and releases available cations such as calcium, magnesium and iron into the water stream. Over time, these elements combine with the dissolved CO2 and form carbonates filling up the empty space (pores) within the rocks.
So water erodes the rock and pulls off elements that naturally combine with CO2 to form carbonates. These are very sticky, and fuse into the basalt pores.
This approach is has folks excited for many reasons:
CO2 fuses with basalt much faster than early models predicted
CO2 (in the form of carbonates) is stored for thousands of years with virtually no chance of breaking out
The basalt + carbonate is stronger than basalt on its own. This makes the formation more stable, a bit like re-pressurizing old oil wells
Learning how mineralization works at scale is useful because it will have other applications, like infusing CO2 into cement
ALL THE OPTIONS
While it’s tempting to rank these storage options from best to worst, that’s actually not useful. What we really want is many storage options — they all have their advantages, and we should learn how to scale all of them.
One really great thing about having many options for storing CO2 is that these different geologic formations — old O&G wells, aquifers, and basalt fields — are spread out in different parts of the world. As we’ll see next week, that means that just about every part of the globe has access to storage, which will make it easier to scale up carbon capture.
The opinions in this article are my own and do not reflect the position of my employer, Bain & Company
Cars and trucks account for 20% of emissions in most countries. If half of the fleet uses recycled fuels, and the remaining half transitions to EVs or hydrogen (a likely scenario according to many forecasters), that means that ~10% of emissions can be covered by recycled fuels — about ~4B tons of CO2 at today’s transport emissions. We’ll be taking at least 40B tons of CO2 out of the air every year once carbon capture tech scales.
This process is called Enhanced Oil Recovery (EOR), and has mostly been used in the Southern United States. Occidental Petroleum is the leading EOR player, and that has put them at the forefront of the largest Direct Air Capture (DAC) plant currently under construction.